As illustrated by the graph below, the spectral characteristics of light reflected from inland waters depend primarily on the relative abundance of three important “optical” constituents:
- Plant pigments (chlorophyll and phycocyanin)
- Colored dissolved organic matter (CDOM)
- Turbidity and suspended matter
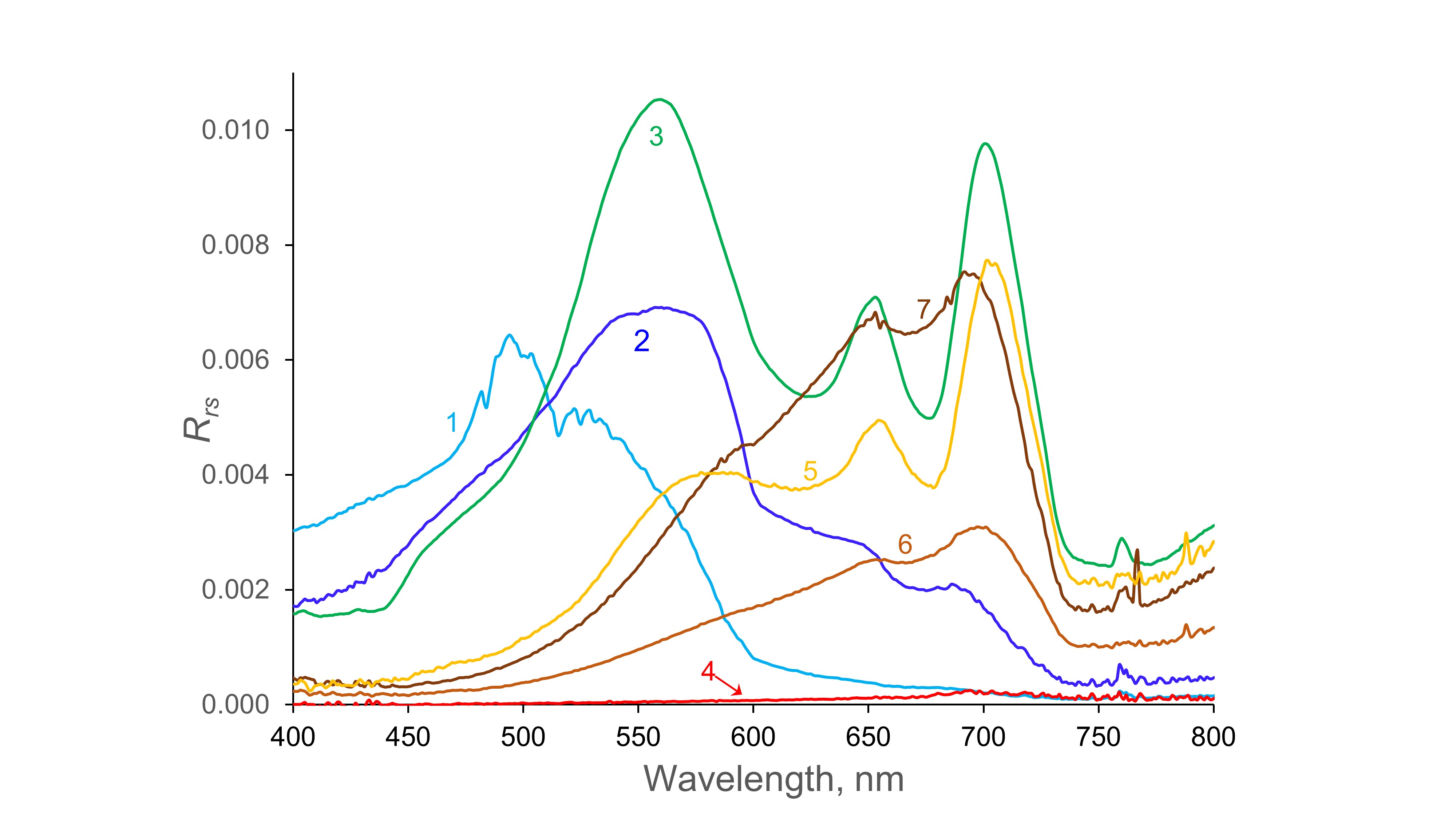
Reflectance spectra for waters dominated by various optical properties:
1. nearly pure water, 2. clear, oligotrophic water, 3. chlorophyll, 4. CDOM, 5. CDOM and chlorophyll, 6 and 7. CDOM and suspended matter.
The spectra in the above figure were obtained by in situ field measurements and represent the fraction of incident light to the lake surface that is reflected back to the atmosphere in the visible and near infrared region (see Burket et al. [2023] for measurement details. Spectrum 1 is for water from a highly oligotrophic, very clear and deep mine pit (Sabin Mine Lake), near Biwabik in northern Minnesota. It has a reflectance maximum near 500 nm, a secondary maximum around 525 nm, and a shape close to that for pure water. Spectrum 2 (Woman Lake, near Hackensack, MN) is a more typical spectrum for low-CDOM, oligotrophic waters. It has a reflectance maximum near 560 nm, similar to that for chlorophyll-dominated, eutrophic Halstead’s Bay (Spectrum 3) of Lake Minnetonka (Hennepin County, MN), but the oligotrophic lake has only a very small reflectance trough at 670 nm (characteristic of chlorophyll absorption), and it lacks the reflectance peak at 705 nm characteristic of scattering by phytoplankton cells. In contrast, Halstead’s Bay has a pronounced trough at 670 nm and peak at 705 nm. The secondary minimum at 620 nm in the Halstead’s Bay spectrum is characteristic of phycocyanin absorbance and indicates that cyanobacteria were present in the lake.
Spectra 4-7 all have low reflectance in the blue region (< 500 nm) because of high CDOM absorbance. The very low spectra for Johnson Bog and Section Eleven Lake (both near Side Lake, MN) in spectrum 4 represent waters optically dominated by very high CDOM (a440 > 20 m-1), which absorbs nearly all incoming light, leaving little to be reflected, even at long wavelengths. Spectrum 5 (Big Sandy Lake, near McGregor, MN) represents a water with both high CDOM and high chlorophyll, resulting in low reflectance in the blue region and the 670 nm trough and 705 nm peak characteristic of chlorophyll. The last two spectra (6 and 7) are for waters with high suspended matter (largely clay and mineral particles) and high CDOM. They have low reflectance at wavelengths < 500 nm, characteristic of CDOM and peaks at 705 nm indicative of light scattering by suspended matter. Both have only small reflectance troughs at 670, indicating that chlorophyll had a small influence on their spectral properties, but Flowage Lake, near McGregor, MN (spectrum 6), has lower suspended solids levels, hence lower reflectance at high wavelengths, than the St. Louis River, near Proctor, MN (spectrum 7).
Satellite sensors that measure the intensity of light reflected from Earth to the sensor in appropriate wavelength ranges can be used to measure these water quality variables. Retrieval of information on the abundance of these constituents in inland waters from satellite sensors typically uses sensor bands that measure characteristic absorption or reflectance features of these constituents and that have minimal interference from other optical properties. For example, retrieval equations for chlorophyll typically use the ratio of the scattering peak for phytoplankton cells at ~ 705 nm and the reflectance trough at ~ 670 nm, which is where peak chlorophyll absorbance occurs. These wavelengths generally are not affected by CDOM absorbance, as shown in the figure by the similarity of the spectra for lakes 3 and 5 in this wavelength region. Marine remote sensing scientists measure chlorophyll using bands related to chlorophyll absorbance in the 450-550 nm region, but CDOM levels are low in the oceans and do not interfere.
The abundant aquatic resources of the Upper Great Lakes region are critically important for recreational activities, agriculture, drinking water supplies and many other economic activities. They also serve as recipients of treated wastewater. The heavy reliance of the region’s economy on its water resources translates into high vulnerability to potential changes in water quality, and human activities can impact surface water quality in many ways. Lake eutrophication (nutrient over-enrichment resulting in excessive production of algae and cyanobacteria) is a pervasive problem in the region. Its primary causes are nutrient inputs from municipal wastewater, urban and agricultural runoff, and near-shore land use. Suspended sediment loadings related to land management practices are a cause of poor water clarity in southern Minnesota; many organic and heavy metal contaminants also are associated with suspended sediment loadings. Mercury pollution is a widespread problem, especially in northern Minnesota, and mercury levels in fish are associated with CDOM levels. Understanding the links between surface water quality and environmental drivers is a major focus of water resources research.
Monitoring surface water quality is a major activity for government water management agencies and many citizen groups. Such efforts provide a vital service for understanding and managing surface waters, but because of cost and human resource limitations, only a small fraction of lakes and rivers can be sampled annually; fewer still can be assessed at frequencies high enough to detect rapid change. Satellite remote sensing can be a cost-effective way to gather spatially comprehensive water quality information.
Long-term water quality information at broad regional scales is a fundamental need for effective environmental planning and management. The extraction of historic and current water quality data from satellite images, coupled with existing data collection, facilitates the development of comprehensive regional databases (e.g., LAGOS) that can be used to evaluate regional differences and trends over time, as well as to evaluate the effects of land-use practices. Results from such analyses can help local and state agencies make informed policy decisions and improve the management of our surface water resources.